Lipid technology for delivery of gene-editing therapies
Gene-editing technology is moving from the research lab to the clinic, one of the most eagerly anticipated biopharma developments in recent history. One of the biggest hurdles to the development of successful gene-editing therapeutics is effective delivery of the large and sensitive components needed to execute the editing functions. Lipid-based nanoparticles present promising solutions to these challenges.
Dr Stephen Burgess; PhD, Head of Nucleic Acid Delivery
WHITEPAPER: Lipid technology for delivery of gene-editing therapies
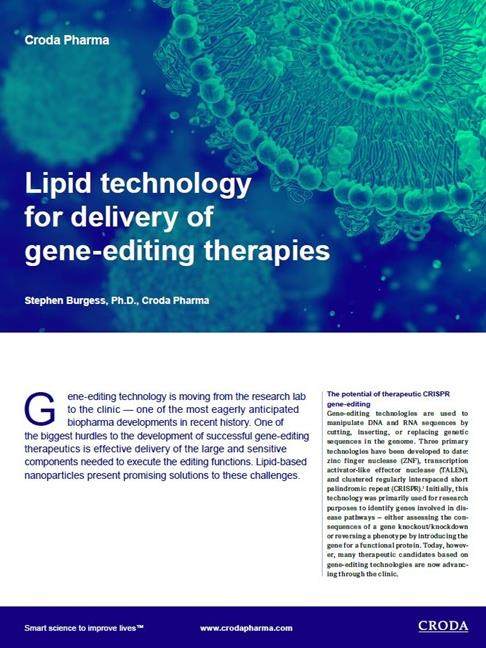
This is an interactive article, simply click the blue words for more information
The potential of therapeutic CRISPR gene editing
Gene-editing technologies are used to manipulate DNA and RNA sequences by cutting, inserting, or replacing genetic sequences in the genome. Three primary technologies have been developed to date: zinc finger nuclease (ZFN), transcription activator-like effector nuclease (TALEN), and clustered regularly interspaced short palindromic repeat (CRISPR) .1 Initially, this technology was primarily used for research purposes to identify genes involved in disease pathways - either assessing the consequences of a gene knockout/knockdown or reversing a phenotype by introducing the gene for a functional protein. Today, however, many therapeutic candidates based on gene-editing technologies are now advancing through the clinic.
The developers of the first version of CRISPR/Cas9 gene-editing technology received the 2020 Nobel Prize in Chemistry for their work. A host of advances have been made since the original CRISPR editing tools were introduced — primarily via genetic engineering — with some of the current candidates leveraging second-generation technology and others relying on third-generation approaches that have addressed some of the earlier issues with the precision and persistence required for therapeutic applications. Further improvements are anticipated as candidates move through preclinical and clinical development and more knowledge is gained.
A number of companies that have been exploring this approach are currently seeing rapid acceleration of their programs. For instance, Verve Therapeutics and Intellia Therapeutics are approaching clinical trials. Many new companies are entering the space as well, quite a number of them focused on in vivo therapies. As gene-editing systems are optimized and become more precise and efficient, the ability to address new and different diseases will become possible, and there will be continued movement toward in vivo therapeutics.
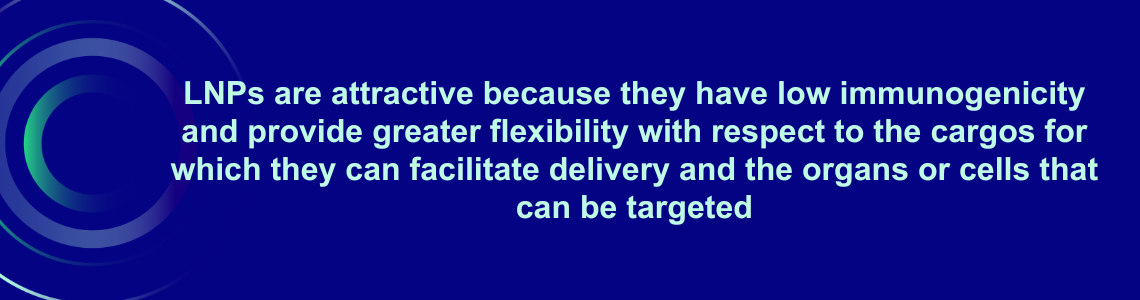
So, why are LNPs attractive delivery vehicles?
Several different approaches have been employed for the delivery of CRISPR gene-editing tools, and they can generally be classified as physical, biological, or chemical2 Physical delivery methods include electroporation, microinjection, and sonoporation. Electroporation can be effective at delivering Cas9 RNP into hard-to-transfect cells, including induced pluripotent stemcells2 It is inefficient for in vivo delivery, however, so most uses have been in ex vivo applications. Microinjection involves injection of the CRISPR components into cells under a microscope and thus has limited throughput. In addition, while there is no cargo size limitation, it does require specialised equipment and expertise and is largely focused on the zygote stage, which is not appropriate for human therapeutics. Sonoporation combines ultrasound with injection and has been investigated in a few development products.
Biological delivery largely involves the use of viral vectors and virus-like particles.2 With viral vector delivery, the CRISPR/Cas9-encoding sequences are integrated into the viral genome, resulting in release of the RNP complex into the targeted cells.1 There are safety concerns (e.g., mutations, carcinogenesis, immune response) and packaging limitations with certain viral vectors. Exosomes (membrane-bound vesicles) are another natural alternative for CRISPR/Cas9 delivery that are being explored because of their biocompatibility and low immunogenicity.
Delivery of CRISPR gene-editing tools can also be achieved with chemical methods, including liposomes, lipid nanoparticles (LNPs), polymeric nanoparticles, gold nanoparticles, and other nanoscalesolutions.1,2 LNPs are attractive because they have low immunogenicity and provide greater flexibility with respect to the cargos for which they can facilitate delivery and the organs or cells that can be targeted.1,3They can also be formulated with biodegradable components as lipid-like nanoparticles (LLNs) with reduced liposome biological toxicity.
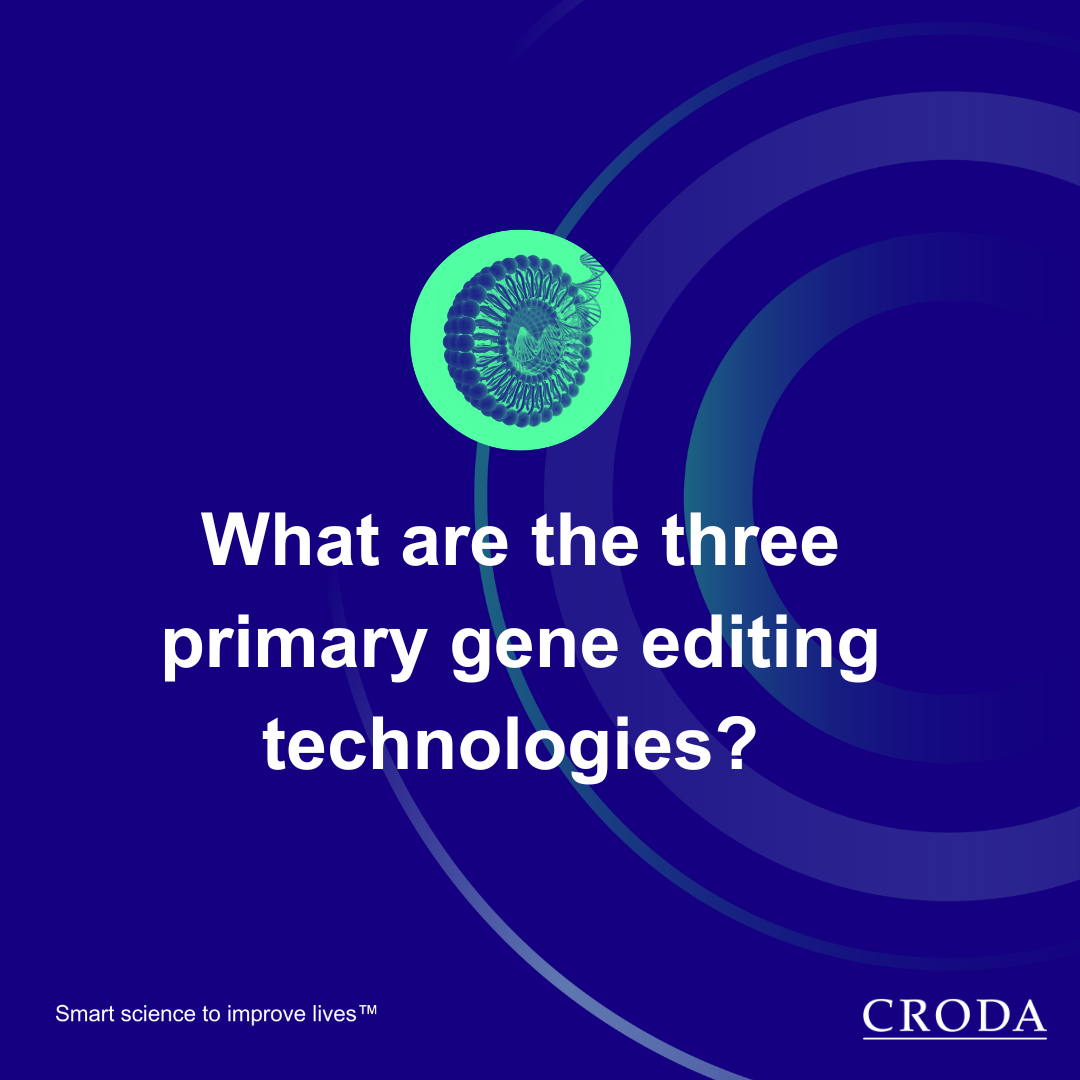
In one recent example, a CRISPR-Cas9/sgRNA system developed as a gene-editing therapy to treat Duchenne muscular dystrophy (DMD) was achieved using a chemically defined LNP solution that enabled safe and repeated injection of the therapy into skeletal muscle tissue in a mouse model.4
Overall, LNPs increase the efficiency of invivo CRISPR/Cas9 component delivery and reduce toxicity compared with viral vector technology while maintaining the necessary precision to achieve therapeutic effects.
What are the packaging, efficiency, and precision challenges?
While LNPs are attractive as delivery vehicles for CRISPR/Cas9 gene-editing components, there are challenges that must be overcome. One difficulty is determining how to package enough material into the LNPs. Research is therefore focused on developing LNP delivery vehicles that can carry larger payloads. Increasing LNP delivery efficiency and precision are other primary goals. With gene editing, it is necessary to deliver the components to a large number of cells; high delivery efficiency is therefore necessary to enable cost-effective doses. Preventing off-target editing is equally crucial.
Can you explain more on LNPs with lipid-coated polymer cores?
Hybrid LNPs present a potential solution. They have solid polymeric cores that sequester genetic material and an external lipid coating comprising lipid components that are biocompatible and make it easier to cross the lipid membranes of cells. They are highly efficient at sequestering and protecting the genetic material and enabling its intercellular uptake. Even the non-natural ionizable lipids, depending on how they are manufactured, are biocompatible and metabolizable and thus do not present toxicity issues. In addition, the polymeric core means that less lipid material is needed, which further reduces the toxicity.
Furthermore, it is possible to tether a targeting agent — a peptide, protein, or antibody — to the lipid to enable the targeting of these therapies to specific areas. The key issue, then, is designing the polymeric core so that it can sequester the large gene-editing components while maintaining the desired delivery efficiencies. Advances in the development of polymeric cores for hybrid LNPs will enable the efficient, targeted delivery of greater quantities of genetic material with reduced toxicity, making polymeric core design a key area of research and development in LNP production.A long-term lipid innovations partner
Croda Pharma is a leading partner for the development of excipients and the supply of high-purity materials for pharmaceutical formulations. We are focused on empowering biologics drug delivery through our adjuvant systems and small molecule, protein, and nucleic acid delivery platforms. With a wide range of solutions for both the human and animal health markets, our pharmaceutical portfolio is unsurpassed in its excellence. Our products, along with in-house formulation and regulatory expertise, allow us to meet the most demanding formulation needs. We are committed to enabling the next generation of drug delivery systems.
Avanti Polar Lipids, now part of Croda Pharma, has been supplying lipids for therapeutic applications, including lipids used for gene therapies, for more than 30 years. Researchers investigating both viral vector and non viral delivery systems have been reliably provided with high-quality, well-characterised materials.In addition to supplying materials currently in use for the delivery of CRISPR/Cas9 gene-editing components, Avanti is pursuing— in cooperation with our customers — efforts to develop innovative lipid products that can address some of the issues that have arisen with LNPs for mRNA vaccines around PEG lipids and other components. Working collaboratively with clients on lipids for these and next-generation gene-editing therapeutics is how Avanti and Croda apply smart science to improve lives.
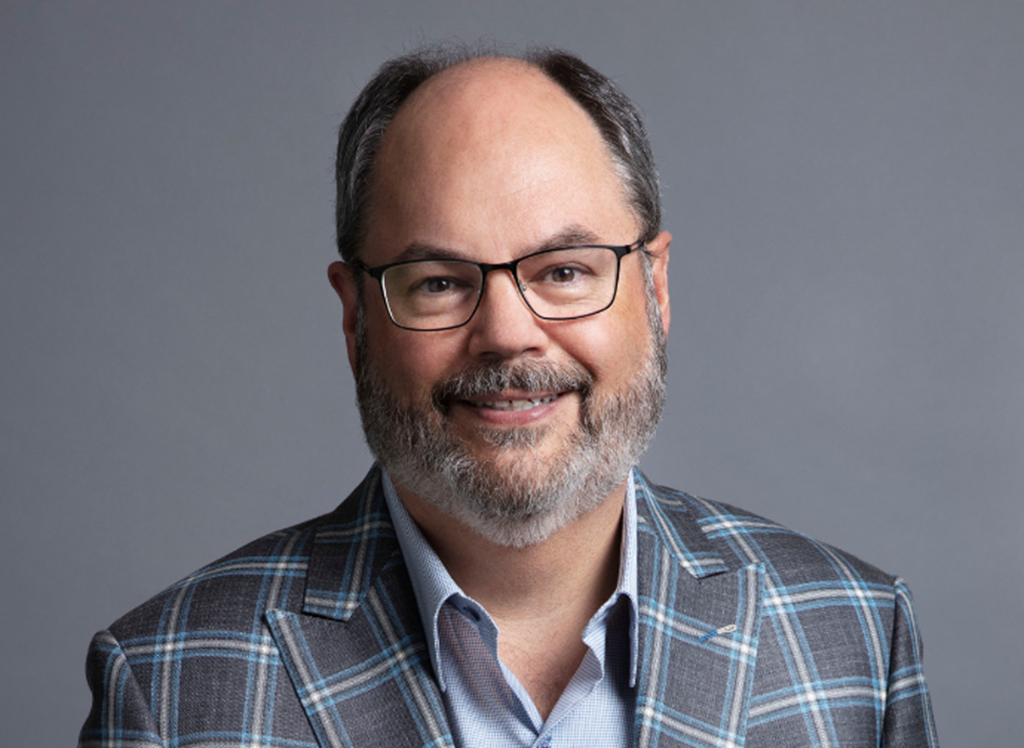
Dr Stephen Burgess
Dr Stephen Burgess is Head of Lipid Technology and Nucleic Acid Delivery. Over the past 30 years, Dr Stephen Burgess has worked with numerous researchers to develop novel lipids and lipid formulations to address specific experimental challenges and improve drug delivery. He has played a key role in a diverse list of projects, including drug delivery development, gene therapy, wound healing, and vaccine development. His background in lipid synthesis, lipid biophysical properties, and membrane dynamics has been instrumental in resolving problems associated with these projects.
References:
1. Duan, Li et al. “Nanoparticle Delivery of CRISPR/Cas9 forGenome Editing.” Front. Genet. 12 May 2021.
2. Taha, Eman A., Joseph Lee, and Akitsu Hotta. “Delivery ofCRISPR-Cas tools for in vivo genome editing therapy: Trends andchallenges.” Journal of Controlled Release. 342: 345–361 (2022).
3. Ross, Colin J. D. et al. “Lipid-Nanoparticle-Based Deliveryof CRISPR/Cas9 Genome-Editing Components.” Mol.Pharmaceutics. 19: 1669–1686 2022.
4. Kenjo, Eriya et al. “Low immunogenicity of LNP allowsrepeated administrations of CRISPR-Cas9 mRNA into skeletalmuscle in mice.” Nature Communications. 12: 7101 (2021).